Sammendrag
Sea-cage salmon farming creates ideal conditions for population growth of the salmon louse Lepeophtheirus salmonis, potentially leading to poor welfare and mortality in farmed salmon and nearby wild salmonids. Frequent delousing treatments are necessary, but current treatments have drawbacks in terms of financial cost, stress to stock, and/or environmental impacts. We tested whether 254 nm ultraviolet-C light (UVC) could function as a new treatment to reduce production of infective copepodids in infested sea-cages. In Experiment 1, we removed mature egg strings from female lice and exposed the egg strings to precise doses of UVC light. A total dose of 0.008 J cm-2 reduced copepodid production by 5 %, while a 95 % reduction occurred at 0.09 J cm-2. In Experiment 2, we exposed salmon with attached adult lice to UVC light while they swam freely in tanks over a 6 day period, achieving a dose of ~0.1 J cm-2. The treatment resulted in a 99 % reduction in copepodid production relative to control groups. However, UVC negatively impacted fish welfare, causing higher rates of cataracts and skin irritation. In Experiment 3, we tested the sensitivity of fish (without lice) to increasing doses of UVC light, and found that minor skin injuries occurred at >60 % effective doses, while cataracts began to develop at very low doses. We conclude that UVC should only be used with caution, either for treatment of waste water to prevent louse eggs and larvae entering the environment (e.g. after delousing), or for short periods of time in-cage to suppress lice reproduction until fish are harvested.
1 - Introduction
1.1 - Background
Parasitism of farmed Atlantic salmon (Salmo salar) by sea lice has severe impacts on production and welfare of both farmed and wild salmonids. In particular, the salmon louse Lepeophtheirus salmonis is perhaps the most intractable issue facing salmon aquaculture in the northern hemisphere. Lice abundance is amplified by the high densities of salmon hosts in sea-cages, causing spill-back onto wild salmon and ongoing reinfestation of farmed salmon. High lice densities at salmon farms reduce productivity and welfare of stock (Øverli et al., 2014) and are implicated in declines in adjacent wild salmon populations (Krkošek et al., 2013). Accordingly, regular lice control is necessary to suppress lice populations, but many existing delousing treatments have significant drawbacks in terms of cost and animal welfare. New lice control methods are required for ongoing suppression of lice populations at salmon farms.
Ultraviolet (UV) light treatments may have potential to reduce lice population growth, either by causing direct mortality of attached lice stages, or by rendering lice eggs or larvae inviable. Ultraviolet wavelength radiation (UVA: 320–400 nm; UVB: 280–320 nm; UVC: 200–280 nm) has a long history of antimicrobial use for disinfection of substrates such as air, water and food preparation surfaces (Bintsis et al., 2000; Severin, 1980), but has not yet been employed in the context of ectoparasite treatment. UV radiation damages nucleic acids by causing strand breaks (Friedberg et al., 2005). These mutations can block DNA transcription and lead to aberrant cell behaviour and/or loss of fidelity during replication. Cellular mechanisms exist to mitigate DNA damage, either by directly reversing modifications or by excising damaged elements (Friedberg et al., 2005), but sufficient exposure can exceed repair mechanisms and lead to cell death. UVC is the most widely used wavelength, as it is relatively inexpensive to produce using low pressure mercury vapor lamps with a peak 254 nm wavelength that is close to the maximal absorption spectrum of DNA, allowing lethal DNA damage to be induced with high efficiency (Cleaver, 2006; Friedberg et al., 2005).
1.2 - Project scope and aims
The aim of the project was to test a range of doses of 254 nm UVC light to determine if they reduced production of copepodids from salmon louse egg strings. We conducted a multi-factorial experiment in which we varied light intensity, exposure duration, and number of exposures, to investigate the threshold at which the cumulative duration of multiple short exposures affected copepodid production. Specifically, we aimed to (1) quantify effects of specific doses of UVC light on egg string hatching rates and copepodid production; (2) conduct a proof-of-concept trial to test whether the same effect occurs when egg strings are attached to female lice on host salmon in a tank environment; (3) assess potential impacts of UVC exposure on host fish welfare; (4) discuss opportunities and challenges for industry deployment of the method.
1.3 - Project organisation
Project group: Frode Oppedal (project manager) and Samantha Bui from the Institute of Marine Research, and Luke Barrett, Cassandra Pert and Tim Dempster from the University of Melbourne, Australia.
Reference group: Solveig Willis (Salmon Group AS, later Pixelwerk AS) and Geir Magne Knutsen (Bremnes Seashore). Kjell Maroni represented FHF as an observer.
2 - Methods
We conducted 3 experiments addressing project aims 1-3. Experiment 1 involved the exposure of egg strings to precise doses of UVC under controlled conditions. This provided data on the dose-response relationship between UVC and copepodid production from exposed egg strings, and identified target doses for subsequent experiments. In Experiment 2, we validated the UVC sterilisation method by applying the 95% effective dose (identified in Experiment 1) to gravid adult female sea lice attached to free swimming salmon in tanks. Experiment 3 was focused on documenting effects of UVC exposure on welfare of salmon by tracking welfare metrics across a range of UVC doses (in the absence of sea lice). This allowed us to determine whether there is a safe short-term UVC exposure level for salmon.
All experiments were conducted at the Matre Research Station, Institute of Marine Research. Experiment 1 was conducted in November-December 2017, Experiment 2 in March-May 2018, and Experiment 3 in March-April 2019.
2.1 - Experiment 1: efficacy against lice egg strings
2.1.1 - Procurement of egg strings
Adult female lice were collected from salmon at research sea-cages in the area and transferred to hosts held in indoor tanks (Ø = 3 m, volume = 5 m3) at 15°C, 34 ‰ salinity. O2 saturation was maintained above 85 %. Over the course of this experiment, lice egg strings were obtained from this population when required.
At this temperature, females extrude a set of egg strings approximately every 5 days, with hatching time of eggs correlated with time of extrusion (Hamre et al., 2019). Female lice produce two egg strings concurrently, and no previous studies have reported differences between egg strings within a pair. Therefore, we used a matched pair design in that one egg string served as the control, while the other underwent the treatment.
Prior to a treatment run, egg strings were collected from adult females and placed into individual incubation wells (Hamre et al., 2009). The incubators were supplied with flowing seawater from the same header tank that supplied stock holding tank. An egg string pair was only collected if both egg strings appeared to be the same length to the naked eye.
2.1.2 - UVC treatment
UVC exposures were delivered in a rectangular raceway (260 cm × 41 cm × 16 cm, 170 l). Three 11 W mercury UVC lamps (254 nm) were located at one end of the raceway, housed within an open box lined with reflective material to direct light toward the raceway. Egg strings were exposed to timed bursts of UVC light using an opaque plastic divider that could be manually raised and lowered. No UVC light was detected in the raceway when the divider was lowered.
To test the effect of UVC on the hatching success of salmon lice egg strings, egg strings were exposed to UVC at different intensities, exposure frequencies (10, 100 and 250 exposures) and exposure durations (2 and 5 seconds). UVC intensity was adjusted by changing the distance between the light source and the egg string (eight distances from 10 cm to 200 cm). Together, the design provided 48 treatment combinations (8 intensities × 3 exposure frequencies × 2 exposure durations), with 5-6 replicate egg string pairs per treatment combination.
UVC wavelength light is rapidly attenuated by seawater according to a negative exponential function. To quantify UVC light attenuation, and thus estimate UVC intensity at a given distance from the light source, we used a germicidal UVC sensor (PMA2122-WP, Solar Light, PA; sensitivity: 254 nm, 10 nm bandwidth; range: 0-2000 µW cm-2) and datalogging radiometer (PMA2100, Solar Light, PA) to measure the maximum UVC intensity at 0.1 m intervals from the light source from 0 to 2 m in a raceway filled with seawater (15°C temperature, 34.2 ‰ salinity, 8.7 mg/L oxygen). At each distance, the sensor was placed in the middle of the raceway and aligned to obtain the maximum intensity measurement. Measured intensity ranged from 459 µW cm-2 at 10 cm to 0.17 µW cm-2 at 200 cm.
The raceway was filled with temperature-controlled water from the holding tank prior to each trial (15.1°C ± 0.2, 8.9 mg/L O2, 34.2‰ salinity). One egg string from each pair was placed in the raceway at one of eight distances from the light source. 24 egg strings could be treated simultaneously, with three egg strings at each of the eight distances. At each distance, egg strings were randomly allocated to the left, right or middle of the raceway. The location of each egg string was noted so that the corresponding control egg string in each pair could be placed in the same location for the subsequent trial.
Once the egg strings were in place, UVC lamps were switched on with the divider lowered. After waiting 15 min to ensure that the lamps had reached their full output, the divider was repeatedly raised to expose the egg strings for the chosen duration (2 or 5 sec) with a period of 2 sec between each exposure. After 10, 100 or 250 exposures, the egg strings were removed and placed in individual incubation wells (one egg string per well). The strongest treatment combination—250 × 5 sec exposures at 10 cm distance from the lamp array—provided a cumulative exposure of 0.57 J cm-2.
The process was then repeated with the lamps switched off to apply the control treatment (using the second egg string from each female). To avoid confounding temporal effects, the trial order (treatment-control or control-treatment) was randomly assigned throughout the experiment, and both egg strings from each female were treated on the same day.
L. salmonis has three planktonic, free-swimming larval stages: eggs hatch into the planktonic nauplius I, then shortly after moult into nauplius II and then the infective copepodid stage. Therefore survival after 2 moults (i.e. from hatching to copepodid) indicates a viable, infective individual. We monitored the incubation wells every day after treatment and recorded the date of first hatching for each egg string. The number of living copepodids was measured 3-8 days after the first hatching date, as all lice should have reached the copepodid stage by day 3 and would still be viable up to 8 days at 15 °C (R. Skern-Mauritzen, unpublished data).
As there is considerable variation in the quality of egg string pairs across females and batches, and it is difficult to detect treatment effects on egg strings that would have had low hatching success regardless of treatment, we omitted data from 23/240 egg string pairs in which the control egg string produced <10 copepodids.
2.2 - Experiment 2: efficacy against lice egg strings on host fish
2.2.1 - Study animals
Six cylindrical tanks (3 m diameter, 5 m3) were stocked with 160 post-smolt Atlantic salmon (mean ± SD: 240 ± 10 g) per tank. Tanks were supplied with 100 ± 2 l min-1 filtered seawater at 15 ± 0.2 °C and salinity 34.1 ± 0.2 ‰, with oxygen saturation maintained above 80 %. Automatic feeders delivered feed to satiation throughout daylight hours (4.5 mm standard feed pellets: Skretting, Norway).
Wild adult female lice were collected from a salmon farm and transferred to salmon in tanks to create a stock population. Egg strings were harvested and incubated to produce infective copepodid larvae, which were then added to tanks to infect salmon for the experiment. During infection, tank flow was shut off and 3200 copepodids per tank (20 copepodids per fish) were added, with 45 min allowed for attachment before reinstating water flow. Oxygen saturation did not drop below 60 % during the infection procedure. The infestation resulted in a mean density (± SE) of 1.2 ± 0.2 adult female lice fish-1 in treatment tanks and 1.0 ± 0.2 adult female lice fish-1 in control tanks at the time of egg string sampling.
Effects of UVC exposure on fish are not well known, as UVC radiation does not occur naturally in aquatic environments. However, skin damage has been reported in fish after excessive UVA/UVB exposure (McArdle and Bullock, 1987; Sweet et al., 2012), and given that UVC affects DNA via similar mechanisms to UVB (Friedberg et al., 2005), it is likely that UVC will have similar effects at sufficient doses. Furthermore, as this trial was intended to span more than the developmental period of an egg string at this temperature, the fish would be exposed to a higher cumulative UVC dose than any given egg string. Accordingly, we first conducted a pilot study with a lower total dose (~0.1 J cm-2 over 10 days), and only proceeded to the full dose after observing no welfare issues for the fish.
2.2.2 - UVC treatment
The exposure regime commenced 31 days after infestation to allow time for female lice to mature and produce egg strings. The UVC dose was delivered via low pressure mercury vapor lamps producing peak intensity at 254 nm (Planet Lighting Pty Ltd, Australia) for 10 days. Three of six tanks were randomly selected to receive the UVC treatment (hereafter ‘UVC’ group), with three 40 W lamps hung vertically in a triangular array around the centre of each tank. During the first week of the trial, each lamp was enclosed within a 30 cm diameter cylindrical mesh cage to prevent fish being exposed to strong irradiation (>490 µW cm-2). The remaining three tanks did not receive UVC treatment (hereafter ‘control’ group) and instead contained empty mesh cages in an identical array to function as procedural controls. Fish were monitored daily throughout the experiment and any sick or injured individuals were euthanised immediately. After six days, superficial skin injuries from contact with the cages became apparent, so the cages were removed from both control and UVC tanks for the second week of the trial, with unpowered lamps acting as procedural controls in the control group tanks.
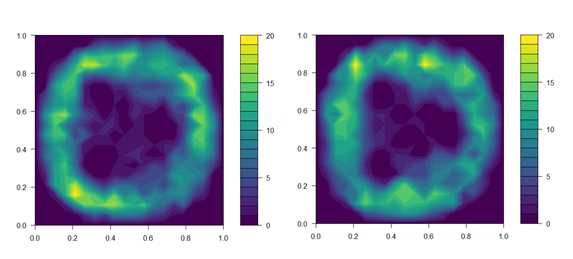
Figure 1. Distribution of fish in treatment tanks with UVC lamps powered off (top panel) and on (bottom panel). Data on fish position is the sum of fish in all three UVC tanks over the first 4 days of the trial. Fish position was noted immediately before the daily UVC exposure began, and again 15 min after UVC lamps were switched on.
We aimed to expose lice egg strings to the 95 % effective dose of 0.1 J cm-2 estimated in Experiment 1. To calibrate the exposure regime to achieve the desired dose, the UVC intensity delivered by the 40 W lamps was measured according to: (a) increasing time from start-up; and (b) distance through the water column. A 20 × 20 cell grid was overlaid over top-down images of the tank, and the maximum irradiance at each cell estimated based on the distance from the nearest lamp. Prior to commencing the exposure regime, the distribution of individual fish within all three treatment tanks was recorded and related to the estimated irradiance at the corresponding grid cells (Fig. 1). This allowed calculation of the UVC intensity received by individuals, and thus, estimation of an exposure regime that would provide the effective dose to both sides of the fish over the developmental period of an extruded egg string. The direction of tank flow was reversed each day to encourage redistribution of fish and ensure exposure of both sides of the body. Initial dosage estimates were further validated over the first four days of the exposure regime by collecting additional data on fish position. We did not account for shading of individuals by those closer to the lamp, so actual doses received will likely be marginally lower than estimated.
2.2.3 - Egg string sampling and fish welfare assessments
Collection of egg strings for incubation took place 42 days after infestation and 11-12 days after the UVC exposure treatments were concluded, coinciding approximately with the fourth egg string pair produced by the female lice and following 11-12 daily UVC exposures. Before sampling, the tank water level was lowered and a light dose of sedative added (0.3 g 100 l-1 metomidate hydrochloride; Aquacalm) before netting randomly-selected fish into 500 L bins containing a full dose of the same sedative (1 g 100 l-1). Tanks were sampled one at a time in random order.
Sedated fish were checked for the presence of adult female lice with egg strings, and up to 24 egg string pairs per tank (range 5-24, mean 19.7 pairs) were transferred to incubators. We euthanised the fish with a blow to the head before conducting full lice counts and welfare assessments on the first 20 fish sampled from each tank (including fin, skin and eye condition) according to the Salmon Welfare Index Model v1.1 (SWIM: Stien et al., 2013). SWIM assessments were also done on a subset of fish after six daily exposures to check for any early welfare effects.
Fish welfare assessments also included behavioural monitoring. Counts of rubbing and jumping behaviours occurring in each tank were made using a live top-down video feed during a 10 min monitoring period per tank on two consecutive days (total 20 min per tank). The 10 min monitoring period was divided into two segments—5 min with the UVC lamps turned off and 5 min with the UVC lamps turned on, in randomised order—to assess whether behaviour is influenced by the immediate presence of UVC light or by cumulative symptoms of exposure. In the control tanks, UVC lamps remained off for both 5 min segments.
2.2.4 - Egg string incubation and copepodid counts
Immediately following collection, egg string pairs were incubated in individual incubator wells (Hamre et al., 2009) supplied with flowing filtered seawater at the same salinity and temperature as the experimental tanks (15 °C and salinity 34.1 ‰).
Larval salmon lice must undergo two moults (nauplius I and II) before reaching the infective copepodid stage. Accordingly, we used the number of copepodids produced from an egg string as an indicator of reproductive success. Incubated egg strings were checked for hatching daily throughout incubation, and copepodid larvae were counted 4 days after the first hatching was observed in an incubator well. This was sufficient time for all viable eggs to hatch and for nauplii larvae to moult into the copepodid stage. Egg strings that remained unhatched 9 days after collection were considered non-viable. If live nauplii were observed during a copepodid count, the well was returned to the incubator for an additional day to allow more time for moulting before copepodid counts were recorded.
Egg strings from two adult female lice were omitted from the analysis because they were the only replicates to receive <2 days of exposure. The dose-response model also omitted 10 UVC egg string pairs (14 % of UVC egg string pairs) that did not hatch, as it was not possible to estimate the date of extrusion without a hatching date.
2.3 - Experiment 3: welfare threshold for host fish
2.3.1 - Study animals
One cylindrical tank (5 m diameter, 20 m3) was stocked with 40 post-smolt Atlantic salmon (1063 ± 146 g). Tanks were supplied with filtered seawater at 9 °C and salinity at 34.1 ‰, with oxygen saturation maintained above 90 %. Fish were fed twice daily to satiation (4.5 mm standard feed pellets: Skretting, Norway). Fish behaviour was monitored twice daily for 10 min for evidence of rubbing or jumping behaviours, both in the UVC treatment tank and monitoring tanks.
2.3.2 - UVC treatment
Exposures were delivered via a single 40 W low pressure mercury vapor lamp hung vertically in the centre of the tank. Fish were kept within 200-250 cm from the light source by means of a plastic-coated steel mesh barrier encircling the centre of the tank. At this range, fish received a measured intensity at 254 nm of 0.5 µW cm-2. Fish swam anti-clockwise throughout the trial so received the dose on the left side only.
The UVC dose was given cumulatively via numerous 15-30 min exposures at 0.5 µW cm-2, with subsamples of 4 fish netted and transferred to 1.5 m3 monitoring tanks as each targeted cumulative dose was reached. The 10 target doses were selected based on the ‘effective doses’ identified in Experiment 1, with fish removed at 10 % effective dose intervals from 0 % (0 J cm-2) to 90 % (0.5 J cm-2) effective doses. 4 fish were also netted into the experimental tank and directly out again, to serve as controls unexposed to UVC doses.
During transfer to monitoring tanks, fish were fully anaesthetised with tricaine methanesulfonate (Finquel: 10 g 100 L-1), checked for obvious injury or illness, and tagged dorsally with colour-coded Floy T-bar tags to allow individual welfare assessments to be matched with UVC dose levels. 1 fish was euthanised during transfer due to a bacterial eye infection.
In the monitoring tanks, fish were fed to satiation via automatic feeders and their welfare monitored daily. At 4-5 days following the last UVC exposure, 2 of the 4 fish at each dose level were euthanised (Finquel: 20 g 100 L-1) and given a formal welfare assessment for skin and eye health.
2.3.3 - Welfare assessments
Welfare was assessed at 4 or 5 days following the last UVC exposure (Sample 1: 2 fish per dose level), and again at 10-23 days following the last UVC exposure (Sample 2: 2 fish per dose level).
Metrics of fish welfare were modified from the SWIM assessment to target UVC-related symptoms based on observations in Experiment 1. We assessed scale loss (1: no scale loss; 2: minor scale loss <10 cm2; 3: extensive scale loss), skin damage consistent with sunburn or physical injury (1: no damage; 2: small old wounds or superficial injury; 3: small open wounds (<1 cm2); 4: many small open wounds or a single large wound; 5: severe condition, potentially life-threatening), and cataract development (1: no cataracts; 2: early stage in a single eye; 3: early stage in both eyes or developed cataract in a single eye; 4: developed cataracts in both eyes; 5: likely blindness).
2.4 - Statistical analyses
In Experiment 1, two variables were recorded to test the effect of UVC treatment: hatching success, taken as a binary response (hatched or not hatched, with any nauplii production being sufficient for a score of ‘hatched’), and developmental success of larvae, taken as the number of larvae per egg string that successfully hatch and moult into the copepodid stage.
Hatching rates in Experiments 1 and 2 were compared across control and treated egg strings using a X2 test of proportions, while developmental success across UVC doses was compared by fitting non-linear dose-response regression models in the drc package for R (Ritz et al., 2015). To avoid overfitting, model functions were ranked by Akaike’s Information Criterion to identify the most parsimonious function. The significance of function parameters was tested using the coeftest() function in the lmtest package (Zeileis and Hothorn, 2002). To account for variance heterogeneity, we used a robust covariance matrix computed by the sandwich package (Ritz et al., 2015; Zeileis, 2004). Some egg strings in Experiment 2 were extruded after the commencement of the exposure regime, and as a result, did not receive the full UVC dose. To account for this, we noted the date of first hatching for incubated egg strings and estimated the likely date of extrusion based on temperature-dependent development times (Samsing et al., 2016).
Numbers of adult lice on UVC and control fish in Experiment 2 were compared using a generalised linear mixed model fitted using glmmTMB (Brooks et al., 2017). Individual fish were treated as replicates, with treatment (UVC, control) as a fixed effect and tank identity as a random effect (nested within treatment) to account for non-independence between tank-mates. As there were more fish with few or no lice than would be expected in a Poisson distribution, we specified a negative binomial model family.
Effects on copepodid production per egg string were compared using a generalised linear mixed effects model fitted using glmmTMB. Female lice were treated as replicates (=incubator well), with treatment (UVC, control) specified as a fixed effect and tank identity as a random effect nested within treatment. We specified a negative binomial model family to account for the large number of egg strings that produced few or no copepodids. As some female lice only had a single egg string attached upon collection, the number of copepodids produced by a female was divided by the number of egg strings to provide an estimate of copepodids produced per egg string.
In Experiment 3, changes in fish welfare with UVC dose were tested with a generalized additive model (GAM) fitted using the mgcv package for R (Wood and Scheipl, 2017).
3 - Results, discussion and conclusion
3.1 - Results
3.1.1 - Experiment 1
The UVC treatment had a strong effect on larval survival, but did not typically cause total hatching failure for salmon louse egg strings; treated egg strings had a 93 % probability of producing at least one nauplius larva (224/240), compared to 95 % (229/240) for control egg strings (test of proportions: X2 = 0.6, df = 1, p = 0.4). However, egg strings exposed to UVC irradiation produced fewer copepodids. This effect exhibited a sigmoidal dose-response relationship that was best fitted by a five-parameter log-logistic function. Model parameters for the slope and inflection point of the response curve were highly significant, indicating a significant dose-dependent effect of UVC exposure on copepodid production. Unexposed egg strings produced 112 ± 4 copepodids per egg string, while the strongest treatment resulted in 9 ± 6 copepodids per egg string. 5 % mortality was achieved at 0.008 J cm-2, with 95 % mortality by 0.09 J cm-2, although modelled estimates for effective doses came with considerable uncertainty at the upper end (Table 1). The 95 % effective dose of 0.09 J cm-2 is equivalent to ~20 mins at a distance of 50 cm (through seawater) from a 40 W low pressure mercury vapor lamp.
Table 1. Predicted effective UVC doses for preventing hatching in 5, 50 and 95 % of lice eggs.
Parameter | Dose (J cm-2) | SE | 95 % CI |
ED5 | 0.008 | 0.001 | 0.005, 0.011 |
ED50 | 0.01 | 0.004 | 0.006, 0.023 |
ED95 | 0.09 | 0.13 | -0.17, 0.35 |
In this study, the total dose was delivered cumulatively by numerous exposures at a given distance. The effect of the dose was not strongly dependent on the specific exposure regimen of the number and duration of individual exposures comprising the total dose. Dose-response functions fitted to data grouped by the number and duration of exposures were similar in inflection points and slopes.
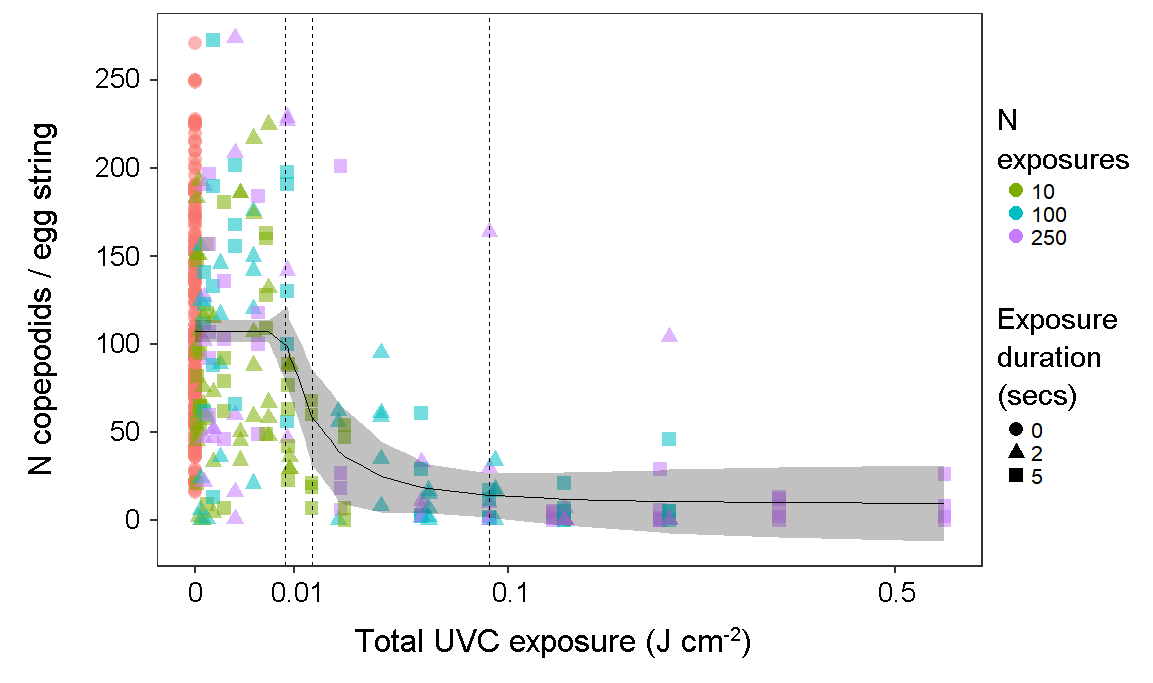
Figure 2. Number of copepodids produced per egg string, according to total UVC exposure (combined light intensity and cumulative exposure duration). (N = 434 egg strings). Symbols are coloured and shaped according to the number and duration of short exposures comprising the cumulative total dose. Vertical dashed lines indicate modelled estimates for 5, 50 and 95 % effective doses (left to right).
3.1.2 - Experiment 2
Numbers of attached lice were highly variable across individual fish and tanks, despite careful quantification of copepodids and all tanks being subject to identical infestation protocols. UVC irradiation was not associated with a lower density of adult lice stages (mean lice per fish ± SE; UVC: 1.2 ± 0.2, Control: 1.0 ± 0.2; negative binomial GLMM: z115 = 0.56, p = 0.57).
86 % of UVC treated egg strings displayed some hatching, whereas all egg strings from control tanks had hatching (N wells = 72 (UVC) + 46 (control), X21 = 5.3, p = 0.02). 25/72 incubator wells in the UVC group underwent at least partial hatching with low moulting success (≤10 copepodids), compared with 1/46 wells in the control group. In many cases, hundreds of nauplii hatched but died, which indicated that UVC exposure affected moulting success of nauplius larvae.
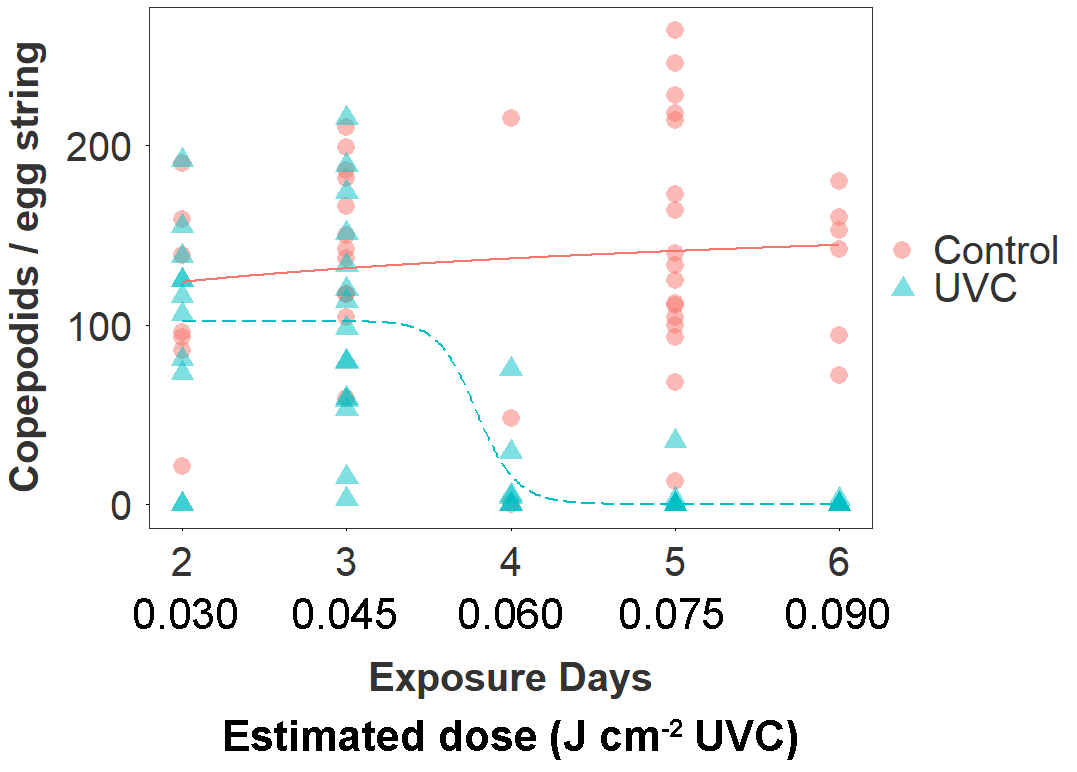
Figure 3. Copepodid production according to treatment group (UVC, Control) and estimated number of days in the between extrusion and collection of the egg string (corresponding to cumulative exposure to the treatment in the UVC group). The estimated date of egg string extrusion was based on the number of days between collection and first hatching. The dose-response relationship is fitted by a three parameter log-logistic function.
The UVC treatment drove a 66 % reduction overall in the number of copepodid larvae produced by treated egg strings (mean copepodids ± SE; UVC: 46 ± 8; Control: 136 ± 9; negative binomial GLMM: z101 = 2.4, p = 0.015). The number of days of exposure to the UVC treatment (estimated from date of first hatching) strongly predicted the magnitude of this effect in the UVC group (Fig. 3). There was a sharp decline in copepodid production in egg strings that had been exposed to the UVC regime for 4 days (60 mins, ~0.06 J cm-2 UVC), and copepodid production was reduced by >99 % after 6 days of exposure (Table 2). The dose-response function estimated a 10 % effective dose for the UVC group at 3.4 days (~0.050 J cm-2), 50 % at 3.7 days (~0.055 J cm-2), and 90 % at 4.0 days (~0.060 J cm-2).
Fish in the UVC group are estimated to have received a cumulative dose of ~0.15 J cm-2 per side by the end of the 10 day trial (0.015 J cm-2 day-1), although this is a mean value and despite efforts to redistribute fish by changing flow direction, some individuals will likely have received a considerably higher or lower dose.
Table 2. Copepodid production per egg string according to estimate dose received by egg string (UVC-treated tanks only)
Est. days exposed | Cumulative dose (J cm-2) | N (adult female lice) | Copepodids per egg string (mean ± SD) |
2 | 0.030 | 11 | 101 ± 60 |
3 | 0.045 | 15 | 103 ± 62 |
4 | 0.060 | 7 | 16 ± 28 |
5 | 0.075 | 9 | 9 ± 21 |
6 | 0.090 | 19 | 0.2 ± 0.7 |
SWIM assessments revealed negative effects among fish exposed to the UVC treatment. UVC treated fish consistently scored more poorly than control fish in fin (lower is better; UVC: 2.3 ± 0.1 vs. Control: 2.0 ± 0.1), skin (4.3 ± 0.1 vs. 1.8 ± 0.1) and eye condition (3.4 ± 0.1 vs. 1.8 ± 0.1). These metrics contributed to a decline in the overall welfare index (OWI: (higher is better) 0.7 ± 0.01 vs. 0.8 ± 0.01). Poorer eye condition scores were due to a higher incidence of early stage cataracts in UVC fish (typically a diffuse 1-3 mm cloudy area in the centre of the eye) and evidence of skin irritation (typically superficial haemorrhaging at the base of the scales).
Fish in the UVC group also exhibited behaviours consistent with skin irritation, including more frequent jumping (2.7 ×) and rubbing against the tank material (32 ×) than the control group (Fig. 4). Bruising from jumping and abrasion from rubbing likely exacerbated the decline in fin and skin condition. Final sampling and euthanasia were conducted as soon as possible after discovering these adverse fish welfare outcomes.
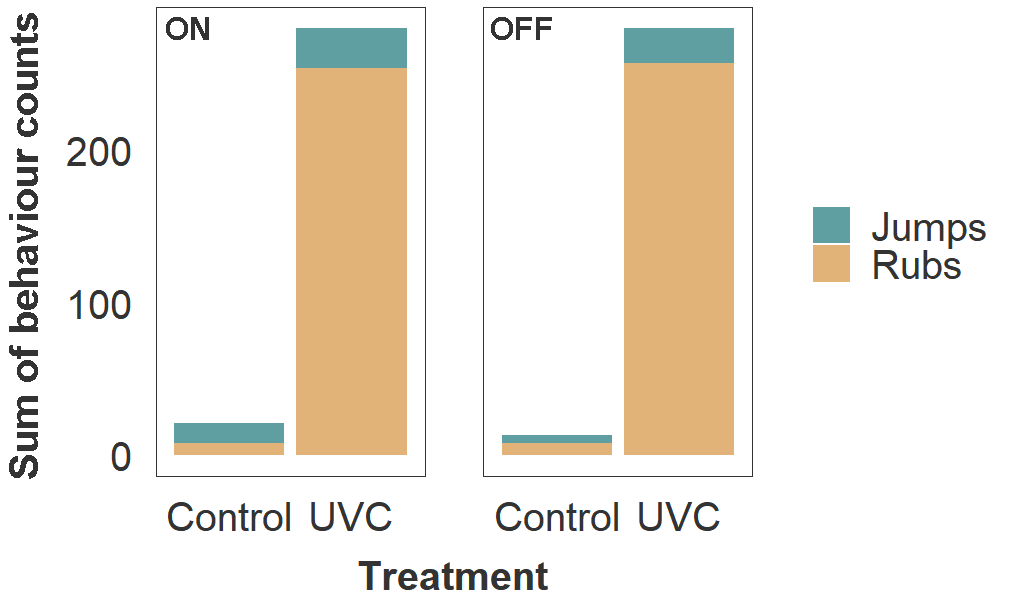
Figure 4. Frequency of rubbing and jumping behaviours in host salmon housed within Control and UVC tanks. Values of stacked bars represent the sum of behaviour counts for all three tanks within each treatment group. Observations were made during a 5 min period per tank, on two consecutive days (total 10 min per tank), while lamps in the UVC tanks were turned on (left panel) and off (right panel).
3.1.3 - Experiment 3
Individuals in Experiment 3 were divided into 10 groups, each exposed to a UVC dose corresponding to 0-90% effective doses estimated by Experiment 1. No signs of irritation, such as rubbing or jumping, were observed during twice-daily behaviour monitoring sessions either in the treatment tank or monitoring tanks. However, formal skin and eye health assessments did indicate that condition decreased with increasing dose (Fig. 5). Worsening of welfare over time was most apparent in skin condition, with all fish exposed to higher dose levels (equivalent to >60 % copepodid reduction) developing minor skin injuries. No fish received a skin condition score of 4 or higher. Scale loss did not appear to be related to UVC exposure and remained approximately constant over time regardless of dose level. Cataract prevalence was highly variable but appeared to increase monotonically with dose level. Of the 26/40 fish that were scored as having cataracts, 13 were in the left eye only, 11 were in both eyes, and 2 were in the right eye only.
Table 3. Dose levels given during Experiment 3, corresponding to percent efficacy against lice egg strings (copepodid reduction).
Copepodid reduction (%) | Cumulative dose (J cm-2) | Day transferred | N exposures (15 min each) |
0 | 0 (control) | 1 | 0 |
10 | 0.009 | 3 | 20 |
20 | 0.010 | 3 | 22 |
30 | 0.011 | 3 | 25 |
40 | 0.012 | 3 | 27 |
50 | 0.014 | 4 | 31 |
60 | 0.017 | 4 | 38 |
70 | 0.021 | 5 | 47 |
80 | 0.029 | 7 | 65 |
90 | 0.051 | 11 | 113 |
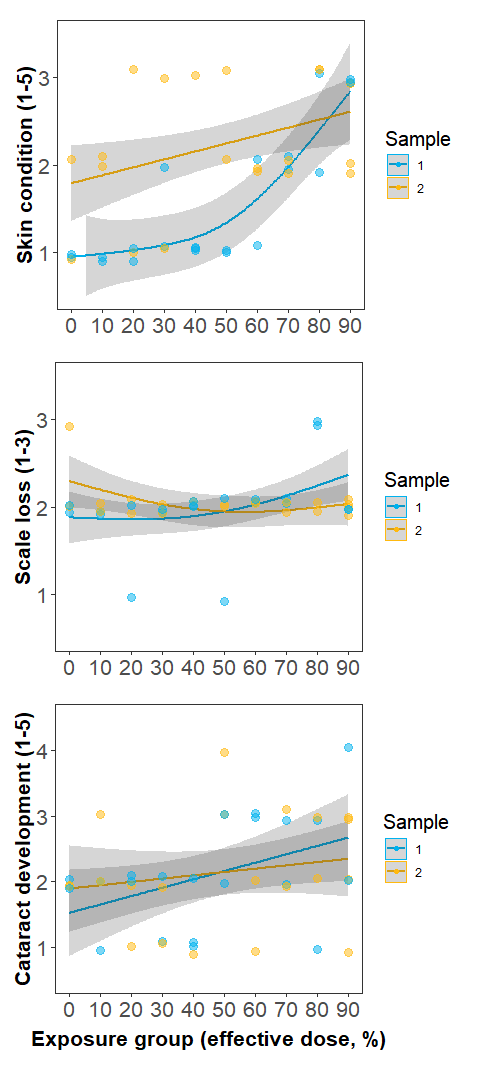
Figure 5. Changes in fish welfare metrics with increasing UVC dose. Sample 1 (blue) was recorded 4-5 days after the final UVC exposure, while Sample 2 was recorded 10-23 days after the final UVC exposure. Minor skin injuries (top panel) became apparent at a ~60 % effective dose in Sample 1, and were still apparent at Sample 2. Scale loss (middle panel) did not appear to be related to UVC exposure, with minor scale loss due to handling, regardless of exposure level. Cataract development (lower panel) increased monotonically with UVC dose.
3.2 - Discussion
3.2.1 - Effects of UVC on salmon louse egg strings
Exposure to UVC wavelength light caused substantial reduction in salmon louse copepodid production in Experiments 1 and 2. The effect correlated with the total dose received and near complete mortality of fertilized eggs was induced at the highest doses (0.1 J cm-2).
Mortality occurred over several stages of egg and larval development. In Experiment 1, 93-95 % of egg strings underwent at least partial hatching regardless of the treatment, but UVC-treated egg strings hatched fewer larvae that successfully moulted into the infective copepodid stage. In Experiments 1 and 2, large numbers of dead nauplii were present in incubator wells with treated egg strings; some died during or shortly after hatching, while others lived for several days as nauplii but did not moult.
The 95 % effective UVC dose in this study (0.09 J cm-2) is higher than the 100 % effective dose for antimicrobial applications (0.00005-0.002 J cm-2: Bintsis et al., 2000) and similar to effective doses for some UV-adapted microbes (Leuko et al., 2011), but much lower than the dose used to kill 100 % of khapra beetle eggs and 98 % of early stage khapra beetle larvae (57 J cm-2: Ghanem and Shamma, 2007). Large variation in effective doses is consistent with the properties of UVC wavelength light and biological tolerance. All else being equal, smaller organisms may be more vulnerable due to reduced attenuation through tissues, but there is also significant variation in evolved radiation tolerance regardless of size. UVC radiation selectively damages DNA via two potentially mutagenic base modifications: cyclobutane pyrimidine dimers (CPDs), and less frequently, pyrimidine (6–4) pyrimidone photoproducts (6–4PP) (Friedberg et al., 2005). Left unchecked, this DNA damage can lead to loss of faithful reproduction (germ cells) or aberrant cell behaviour (somatic cells). Cellular mechanisms exist to mitigate DNA damage, either by directly reversing modifications or by excising damaged elements, but when repair mechanisms are insufficient, cell death will occur. Cell death is catastrophic for single celled organisms and gametes, but in metazoans, damaged epidermal cells can undergo cell suicide and be recycled and replaced, allowing the organism to recover from relatively higher doses. Accordingly, it may be that higher doses are required to kill attached lice stages than early stage zygotes.
3.2.2 - Effects on fish welfare
UV-induced skin damage occurs primarily via DNA strand breaks in epidermal cells. Some amount of DNA damage can be repaired by various mechanisms, but cells with a heavy mutational burdens or genomic instability may instead undergo programmed cell death (apoptosis) in the hours or days following exposure and be replaced (Friedberg et al., 2005). Symptoms of UV damage typically arise in the days following exposure. Histological studies of salmonid skin exposed to UVB irradiation have found increased shedding of the top layers of epidermal cells (Noceda et al., 1997) and intercellular oedema (Bullock and Roberts, 1992). In the present study, epidermal damage in UVC exposed salmon in Experiment 2 prompted excessive rubbing and jumping behaviours in affected fish, which likely led to additional injuries resulting from the physical contact with the tank walls or lamp apparatus. These injuries generally consisted of minor haemorrhaging at the base of the scales, and in the most severe cases, loss of scales and visible scratches or lesions from contact with abrasive surfaces. Mortality rates were high in the UVC group, perhaps driven by a combination of immunosuppression from UV exposure and osmotic stress from skin wounds. Fish did not appear to avoid UVC lamps whether they were switched on or off (Fig. 1), and 2-3 days after the first UVC exposure, rubbing and jumping occurred regardless of whether the lamps were switched on at the time of observation. This indicates that fish do not suffer immediate discomfort from exposure to UVC and accordingly do not attempt to avoid potentially harmful exposure. Although we did not observe behavioural indications of irritation in Experiment 3, the presence of minor scratches on most fish in the 70-90 % dose level groups is consistent with findings from Experiment 2.
Cataracts are common in farmed salmon. There formation has been attributed to a variety of intrinsic and environmental causes, including triploidy, dietary deficiencies, temperature and UV exposure (Ersdal et al., 2001; Sambraus et al., 2017). In this study, a higher frequency of early stage cataracts in the UVC group indicates rapid cataract formation following UVC exposure. Studies in mammals indicate that UV-induced cataract formation stems from DNA breaks in the epithelium (Hightower, 1995; Li and Spector, 1996), with epithelial cell death occurring within 24-48 hours following exposure to UVB, accompanied by changes to gene regulation, ion imbalances and development of tissue opacity. In lake trout, small cataracts became apparent within 48 hours of exposure to UVB doses >0.5 J cm-2, with some recovery in the form of increased clarity observed after 5 days (Cullen and Monteith-Mcmaster, 1993). At higher doses (>1 J cm-2) there was no evidence of recovery after 7 days. Cataracts may occur at even lower doses of UVC relative to UVB, as the shorter wavelength of UVC is more specifically absorbed by the corneal epithelium. In the present study, early cataract formation occurred after 6 daily UVC exposures in Experiment 2 (cumulative ~0.1 J cm-2), with some increase in prevalence and severity observed after an additional 4 days exposure (the time of final sampling). To avoid further animal welfare issues, we did not hold fish to test for recovery of the lens or cornea. In Experiment 3, cataract prevalence in Sample 1 increased gradually with UVC exposure (Fig. 7), with no evidence of recovery after 10-23 days (Sample 2).
3.2.3 - Opportunities and challenges for deployment in the industry
UVC irradiation has immediate applications for aquaculture. Many current delousing methods for salmon farms (such as mechanical and thermal treatments) produce waste water containing live lice, viable egg strings and other pathogens that can enter the environment and increase reinfection risk. Filtering of this waste water is increasingly being employed, but concentrated high doses of UVC irradiation applied to waste water may be a less expensive and more reliable method of preventing viable eggs from entering the environment. UVC treatment may also be applied to other forms of waste water, such as outflow from dead fish collection systems. Light intensities to achieve an effective dose will depend on flow rates and turbidity of water.
Potential applications involving direct exposure of infested fish will have to overcome two major challenges: (1) achieving a sufficient dose cost-effectively given the rapid attenuation of UVC wavelength light in seawater, and (2) supressing lice reproduction without reducing the welfare of salmon over the duration of a grow-out cycle. The first challenge may be best tackled by installing numerous small light sources, for example in the form of a ‘curtain’ of hanging UVC lamps that individuals must pass through while swimming around the cage, or by using lamps in combination with a ‘snorkel’ salmon lice barrier to expose individuals at close range for short durations (Stien et al., 2016). Lower seawater temperatures may favour such an approach, as egg strings develop more slowly and so may be exposed over a longer period of time (Samsing et al., 2016). Underwater lighting is already in widespread use by the salmon industry for photoperiod control to suppress maturation (Oppedal et al., 2007, 2001). The second challenge—side effects on fish—will require careful control of doses to achieve smaller reductions in copepodid production while maintaining acceptable fish welfare. Before any potential deployment in the industry, research is needed to identify how exposure regimes influence side-effects, including long-term symptoms over the course of a grow-out cycle (for example, development of cancer or skin lesions). Further, exposure to UVA and UVB light can interact with other skin conditions, including skin parasites (Ichthyobodo: Bullock, 1985) and existing wounds (Bullock and Roberts, 1992); these interactions should also be tested for UVC.
If effective doses can be given with minimal short-term side effects, there may be cases where suppression of salmon lice reproduction by UVC exposure is a useful method. Specifically, we envision a situation where copepodid production can be suppressed while farms await delousing or harvesting. If it is not possible to suppress lice reproduction with UVC lights within sea-cages without harming salmon, then applications that target lice or egg strings when they have detached from salmon remain possible. Specifically, mechanical and thermal delousing remove lice and either release waste water directly into the sea or attempt to filter all treatment water. UVC treatment of all post-treatment water may prove more cost-effective than filtering technology. Such an application of UVC light may deliver broad benefits by reducing transmission of multiple pathogens (bacteria and viruses) that may also co-occur in treatment waste water.
This project primarily assessed effects of UVC on mortality of lice eggs and larvae, but UVC irradiation at sublethal levels may also have important suppressive effects on adults and therefore lice populations. Sterilised individuals, especially males, can disproportionately impact populations by deceiving conspecifics into investing in infertile matings (Lees et al., 2015). This strategy has been successfully used to control other pest species, including the release of male mosquitos sterilised by radiation to function as ‘ovitraps’ for female mosquitos (Bellini et al., 2013), and may be particularly effective against sea lice as female lice are frequently mate-limited (Cox et al., 2017).
3.3 - Conclusions
Long term suppression of salmon lice populations in the salmon farming industry will depend on multiple complementary strategies, spanning pre-infection prevention to post-infection control. We have demonstrated that sufficient doses of UVC irradiation can dramatically reduce production of infective stages from salmon lice egg strings, and deployment for treatment of delousing system outflow or other waste water from salmon aquaculture could provide immediate benefits. The application of UVC light to lice-infested salmon at via underwater lamps can prevent fertilised salmon lice egg strings from developing into infective stages. The feasibility of this application in full-scale industrial settings will depend on developing an exposure regime that offers cost-effective reductions in lice production with minimal side effects for host fish.
3.4 - Acknowledgments
Approval for ethical use of animals was obtained from the Norwegian government (Mattilsynet FOTS number: 14862). We thank Kjell Maroni (FHF), Geir Magne Knutsen (Bremnes Seashore AS) and Solveig Willis (Salmon Group AS/ Pixelwerk) for discussions relating to potential industrial applications. We thank Sinclair Park (Planet Lighting; planetlighting.com) for discussion and for providing the UVC lamps and Tone Vågseth (IMR), Georgia Macaulay (UoM), Kathy Overton (UoM/IMR), Michael Sievers (IMR) and Thomas Crosbie (UoM) for technical assistance.
4 - Main findings
Description of dose-response relationship for UVC exposure on hatching success of salmon louse egg strings and copepodid production.
Proof-of-concept trial in a tank environment demonstrating that UVC exposure can suppress production of infective louse stages, but with adverse effects on host salmon.
Documented 'welfare threshold' for post-smolt salmon exposed to frequent UVC doses.
Discussed potential for deployment of UVC irradiation in the salmon aquaculture industry.
5 - Deliverables
5.1 - Meetings with the reference group and FHF representative
Phone meetings have been held three times during the project period, where the reference group were informed of new results in the project, and implications discussed from a scientific and industrial perspective.
5.2 - Communication of results: media
Findings will be communicated to Norsk Fiskeoppdrett / kyst.no for production of a popular news article targeted at industry readers.
Bui, S., Oppedal, F., Dempster, T., Pert, C., Barrett, L., 2019. In situ sea lice egg sterilization with UVC light. Rapport fra Havforskningen X-2019 (denne rapport). 30p
5.3 - Communication of results: scientific publications
Manuscripts submitted to peer-reviewed journals in the field of aquaculture research:
Luke T Barrett, Cassandra G Pert, Samantha Bui, Frode Oppedal, Tim Dempster (Submitted) Sterilization of salmon lice eggs with UVC light: a new preventative technique.
Luke T Barrett, Samantha Bui, Frode Oppedal, Tim Dempster (Submitted) Ultraviolet-C irradiation of salmon lice on salmon reduces production of infective stages but has adverse effects on host fish.
6 - References
Bellini, R., Medici, A., Puggioli, A., Balestrino, F., Carrieri, M., 2013. Pilot field trials with Aedes albopictus irradiated sterile males in Italian urban areas. J. Med. Entomol. 50, 317–325.
Bintsis, T., Litopoulou-Tzanetaki, E., Robinson, R.K., 2000. Existing and potential applications of ultraviolet light in the food industry – a critical review. J. Sci. Food Agric. 80, 637–645. https://doi.org/10.1002/(SICI)1097-0010(20000501)80:6<637::AID-JSFA603>3.0.CO;2-1
Brooks, M.E., Kristensen, K., van Benthem, K.J., Magnusson, A., Berg, C.W., Nielsen, A., Skaug, H.J., Maechler, M., Bolker, B.M., 2017. Modeling zero-inflated count data with glmmTMB. bioRxiv 132753. https://doi.org/https://doi.org/10.1101/132753
Bullock, A.M., 1985. The effect of ultravioletB radiation upon the skin of the plaice, Pleuronectes platessa L., infested with the bodonid ectoparasite Ichthyobodo necator (Henneguy, 1883). J. Fish Dis. 8, 547–550. https://doi.org/10.1111/j.1365-2761.1985.tb00970.x
Bullock, A.M., Roberts, R.J., 1992. The influence of ultraviolet-B radiation on the mechanism of wound repair in the skin of the Atlantic salmon, Salmo salar L. J. Fish Dis. 15, 143–152. https://doi.org/10.1111/j.1365-2761.1992.tb00648.x
Cleaver, J.E., 2006. Cells have long experience of dealing with UVC light. Nature 442, 244.
Cox, R., Groner, M.L., Todd, C.D., Gettinby, G., Patanasatienkul, T., Revie, C.W., 2017. Mate limitation in sea lice infesting wild salmon hosts: the influence of parasite sex ratio and aggregation. Ecosphere 8, e02040. https://doi.org/10.1002/ecs2.2040
Cullen, A.P., Monteith-Mcmaster, C.A., 1993. Damage to the rainbow trout (Oncorhyncus mykiss) lens following an acute dose of UVB. Curr. Eye Res. 12, 97–106. https://doi.org/10.3109/02713689308999477
Ersdal, C., Midtlyng, P.J., Jarp, J., 2001. An epidemiological study of cataracts in seawater farmed Atlantic salmon Salmo salar. Dis. Aquat. Organ. 45, 229–236.
Friedberg, E.C., Walker, G.C., Siede, W., Wood, R.D., 2005. DNA repair and mutagenesis, 2nd ed. American Society for Microbiology Press, Washington, DC, DC.
Ghanem, I., Shamma, M., 2007. Effect of non-ionizing radiation (UVC) on the development of Trogoderma granarium Everts. J. Stored Prod. Res. 43, 362–366. https://doi.org/10.1016/j.jspr.2006.09.002
Hamre, L.A., Bui, S., Oppedal, F., Skern-Mauritzen, R. and Dalvin, S., 2019. Development of the salmon louse Lepeophtheirus salmonis parasitic stages in temperatures ranging from 3 to 24 °C. Accepted for publication in Aquaculture Environment Interactions.
Hamre, L.A., Glover, K.A., Nilsen, F., 2009. Establishment and characterisation of salmon louse (Lepeophtheirus salmonis (Krøyer 1837)) laboratory strains. Parasitol. Int. 58, 451–460. https://doi.org/https://doi.org/10.1016/j.parint.2009.08.009
Hightower, K.R., 1995. The role of the lens epithelium in development of UV cataract. Curr. Eye Res. 14, 71–78. https://doi.org/10.3109/02713689508999916
Krkošek, M., Revie, C.W., Gargan, P.G., Skilbrei, O.T., Finstad, B., Todd, C.D., 2013. Impact of parasites on salmon recruitment in the Northeast Atlantic Ocean. Proc. R. Soc. London B Biol. Sci. 280. https://doi.org/10.1098/rspb.2012.2359
Lees, R.S., Gilles, J.R.L., Hendrichs, J., Vreysen, M.J.B., Bourtzis, K., 2015. Back to the future: the sterile insect technique against mosquito disease vectors. Curr. Opin. Insect Sci. 10, 156–162. https://doi.org/https://doi.org/10.1016/j.cois.2015.05.011
Leuko, S., Neilan, B.A., Burns, B.P., Walter, M.R., Rothschild, L.J., 2011. Molecular assessment of UVC radiation-induced DNA damage repair in the stromatolitic halophilic archaeon, Halococcus hamelinensis. J. Photochem. Photobiol. B Biol. 102, 140–145. https://doi.org/10.1016/j.jphotobiol.2010.10.002
Li, W.-C., Spector, A., 1996. Lens epithelial cell apoptosis is an early event in the development of UVB-induced cataract. Free Radic. Biol. Med. 20, 301–311. https://doi.org/https://doi.org/10.1016/0891-5849(96)02050-3
McArdle, J., Bullock, A.M., 1987. Solar ultraviolet radiation as a causal factor of ‘summer syndrome’ in cage-reared Atlantic salmon, Salmo solar L.: a clinical and histopathological study. J. Fish Dis. 10, 255–264. https://doi.org/10.1111/j.1365-2761.1987.tb01070.x
Noceda, C., Sierra, S.G., Martínez, J.L., 1997. Histopathology of UV-B irradiated brown trout Salmo trutta skin. Dis. Aquat. Organ. 31, 103–108. https://doi.org/10.3354/dao031103
Oppedal, F., Juell, J.-E., Johansson, D., 2007. Thermo- and photoregulatory swimming behaviour of caged Atlantic salmon: implications for photoperiod management and fish welfare. Aquaculture 265, 70–81. https://doi.org/https://doi.org/10.1016/j.aquaculture.2007.01.050
Oppedal, F., Taranger, G.L., Juell, J.-E., Hansen, T., 2001. Growth, osmoregulation and sexual maturation of underyearling Atlantic salmon smolt Salmo salar L. exposed to different intensities of continuous light in sea cages. Aquac. Res. 30, 491–499. https://doi.org/10.1046/j.1365-2109.1999.00362.x
Øverli, Ø., Nordgreen, J., Mejdell, C.M., Janczak, A.M., Kittilsen, S., Johansen, I.B., Horsberg, T.E., 2014. Ectoparasitic sea lice (Lepeophtheirus salmonis) affect behavior and brain serotonergic activity in Atlantic salmon (Salmo salar L.): perspectives on animal welfare. Physiol. Behav. 132, 44–50.
Overton, K., Dempster, T., Oppedal, F., Kristiansen, T., Gismervik, K., Stien, L., 2018. Delousing operations and salmon mortality in Norwegian aquaculture: a review. Rev. Aquac. https://doi.org/10.1111/raq.12299
Ritz, C., Baty, F., Streibig, J.C., Gerhard, D., 2015. Dose-response analysis using R. PLoS One 10, 1–13. https://doi.org/10.1371/journal.pone.0146021
Sambraus, F., Fjelldal, P.G., Remø, S.C., Hevrøy, E.M., Nilsen, T.O., Thorsen, A., Hansen, T.J., Waagbø, R., 2017. Water temperature and dietary histidine affect cataract formation in Atlantic salmon (Salmo salar L.) diploid and triploid yearling smolt. J. Fish Dis. 40, 1195–1212. https://doi.org/10.1111/jfd.12594
Samsing, F., Oppedal, F., Dalvin, S., Johnsen, I., Vågseth, T., Dempster, T., 2016. Salmon lice (Lepeophtheirus salmonis) development times, body size, and reproductive outputs follow universal models of temperature dependence. Can. J. Fish. Aquat. Sci. 73, 1841–1851. https://doi.org/10.1139/cjfas-2016-0050
Severin, B.F., 1980. Disinfection of municipal wastewater effluents with ultraviolet light. J. Water Pollut. Control Fed. 52, 2007-2018 (12).
Stien, L.H., Bracke, M.B.M., Folkedal, O., Nilsson, J., Oppedal, F., Torgersen, T., Kittilsen, S., Midtlyng, P.J., Vindas, M.A., Øverli, Ø., Kristiansen, T.S., 2013. Salmon Welfare Index Model (SWIM 1.0): a semantic model for overall welfare assessment of caged Atlantic salmon: review of the selected welfare indicators and model presentation. Rev. Aquac. 5, 33–57. https://doi.org/10.1111/j.1753-5131.2012.01083.x
Stien, L.H., Dempster, T., Bui, S., Glaropoulos, A., Fosseidengen, J.E., Wright, D.W., Oppedal, F., 2016. ‘Snorkel’ sea lice barrier technology reduces sea lice loads on harvest-sized Atlantic salmon with minimal welfare impacts. Aquaculture 458, 29–37. https://doi.org/10.1016/j.aquaculture.2016.02.014
Sweet, M., Kirkham, N., Bendall, M., Currey, L., Bythell, J., Heupel, M., 2012. Evidence of melanoma in wild marine fish populations. PLoS One 7. https://doi.org/10.1371/journal.pone.0041989
Wood, S., Scheipl, F., 2017. gamm4: Generalized Additive Mixed Models using “mgcv” and “lme4.”
Zeileis, A., 2004. Econometric computing with HC and HAC covariance matrix estimators. J. Stat. Softw. 11, 1–17.
Zeileis, A., Hothorn, T., 2002. Diagnostic checking in regression relationships. R News 2, 7–10.